Autonomous driving is fast becoming a reality. Increasing driving assistance and safety features such as adaptive cruise control and lane departure warnings are already becoming commonplace. Predictive ride technology is being introduced whereby the car senses the state of the road ahead and adjusts the ride settings to maximize comfort and reduce travel sickness when driving over uneven surfaces.
McKinsey projects that by 2030 up to 15% of vehicles sold will be fully autonomous. For passengers, that is likely to mean a smooth and comfortable driverless experience – but this pushes the need to achieve all the external connectivity to meet lifestyle and/or workplace requirements.
Connected cars
In-vehicle networks, infotainment, and safety applications will generate and consume vast over-the-air real-time data streams at speeds of up to 24 Gpbs, thanks to wireless communication and 5G cellular networks. Analysts envision that connected cars will send 25 gigabytes of data to the cloud every hour, using analytics to fuel and optimize complex, interdependent applications, making driving safer for everyone.
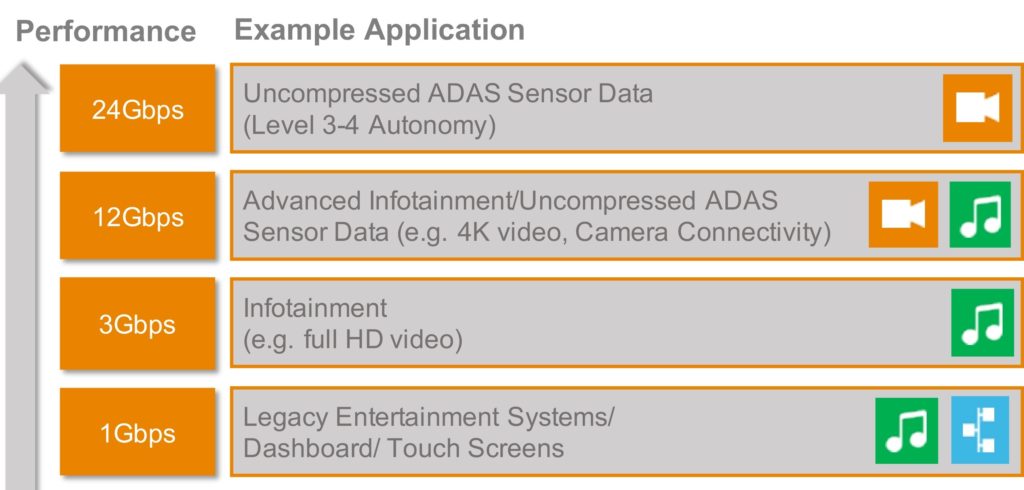
Key enablers of this rapid market and technology transformation are sophisticated sensor, antenna, and data connectivity technologies that work together. These components sense the environment, within and outside the vehicle, and receive, act upon, and transmit data in real-time to devices throughout the vehicle and in the physical world the vehicle navigates.
Obviously, achieving this vision requires sustained technology innovation. Automotive manufacturers and partners need to collaborate on developing innovative solutions that leverage vast data streams and enable real-time analytics; integrate a variety of devices and chip protocols; and address significant challenges such as ensuring no electromagnetic interference (EMI), low signal attenuation, and galvanic isolation. In addition, they must be light weight to accommodate interdependent systems and preserve vehicle acceleration.
Connected car manufacturers want to make sure they can meet the requirements of next-generation architectures and the most critical enablers to power autonomous driving. They will need to consider architecture, high speed data, external connectivity, reliability, safety and design.
New vehicles will have architectures that organize high-performance clusters in functional domains. These service-oriented functional domains will be connected hierarchically in a high-speed data backbone structure, and they will group sensors and actuators. Autonomous driving will increasingly demand highly reliable network-based structures, leading to the development of redundant, real-time architectures.
The number of high-speed data nodes will skyrocket in new car architectures, as vehicles house high-speed computers and an increasing number of ADAS sensors, such as high-resolution stereo and mono cameras; RADAR and LIDAR; and future human-machine interfaces, such as large 4K-8K screens or head-up displays. The increase in data nodes will lead to a corresponding growth in net data payloads for each link, which will vary depending on their throughput requirements. Multiple parallel and bidirectional data streams will need to be managed in switched networks and provide sufficient safety margin. To meet all these requirements, manufacturers should plan on designing flexible architectures that accommodate increasing bandwidths for point-to-point data pipes and distributed network structures.
External connectivity
Vehicle-to-everything (V2X) applications, including safety applications, will need to capture comprehensive and complete real-time data on the surrounding environment. V2X communications is radio-based. Sensors around the car, such as those in lights, signs, and tolls, communicate their status to car systems, enabling connected cars to identify dangerous situations proactively and swiftly.
To enable fully autonomous driving, however, V2X data must be captured and integrated in real-time, so that the car driver or autopilot system can respond almost instantly to hazards. Current cellular radio standards aren’t sufficient to meet this requirement for all required scenarios. However, DSRC, as well as the forthcoming 5G mobile network, have the potential to meet the industry’s low latency requirements and fully realize the benefits of V2X technology.
Autonomous driving requires highly reliable sensing and data transmission in a wide array of conditions. Data connectivity solutions must be robust, automotive-grade systems that will provide continuous fail-safe operations across thousands of hours and harsh environments. Partners should take a holistic approach to designing connectivity solutions, considering mechanical and electrical system design, aligning process engineering and automation solutions, and using new manufacturing procedures.
Connectivity solutions for safety applications must also ensure data integrity. To protect human lives, manufacturers should ensure solutions transport data safely on the physical layer and protect against any possible corruption that could occur in a noisy electromagnetic environment. Systems should be scalable, modular, and offer EMI-immune options. Car manufacturers will want interchangeable shielded and unshielded solutions, as well as fiber optics communications solutions that pose low or no EMI risks.
Connected car systems are lightweighting and miniaturizing to make the most of automobiles’ constrained physical footprint. As connected cars become powerful, mobile data processing networks with high-performance computers and centralized domain clusters, they will have more sensors than ever and longer link lengths. Again, platforms should be scalable and modular, enabling partners to customize components to meet OEM demands. These platforms should support hybrid solutions with high- and low-speed data or power delivery ports, with unshielded or shielded variants to meet different water protection classification requirements.
Data Speed Requirements
Connectivity solutions aren’t one-sized-fits-all: In-vehicle networks, infotainment, and safety applications have different needs for data processing, latency, and redundancy. Looking at these needs more specifically, in-vehicle networks, such as apps, traffic reports, and vehicle health updates, require speeds of up to 150Mbps, whereas legacy entertainment systems, dashboards, and touch screens require speeds of up to 1 Gbps. In-vehicle network links typically leverage the automotive Ethernet to transmit medium-sized data volumes with low latencies cost-effectively over distributed network architectures. Most legacy systems that require high-speed connectivity use point-to-point connections and are thus less scalable. In addition, these applications are derived from existing consumer applications and are based on proprietary standards and are costlier to implement, maintain, and enhance.
Infotainment applications, such as those with full HD video, will require speeds of up to 3 Gbps. Advanced infotainment, such as apps with 4K video and camera connectivity, or apps using uncompressed ADAS sensor data, will require speeds of up to 12 Gbps. With high data rates, infotainment links must be high-performance and provide time-synchronous data streams on multiple devices. Currently, they are typically point-to-point connections. In the future, they may use open protocols to transmit across automotive networks.
And finally, level 3 and Level 4 autonomous driving and safety apps using uncompressed ADAS sensor data will require speeds of up to 24 Gbps. These links must provide real-time, reliable computing of high data volumes to ensure high functional safety levels. Data volumes are so high because safety applications use high-resolution camera imagery that is delivered uncompressed, with high refresh rates. Today, safety links are large point-to-point data pipes that use proprietary transmission technologies. In the future, functional safety architectures will use fail-safe and redundant topologies, such as those based on ring bus systems. Real-time network technologies will ensure data high availability with low latencies, potentially leveraging open-protocol, time-sensitive networking to achieve this goal.
It is clear that functional and safety needs define link types and requirements. Similarly, link characteristics define data connectors, and attributes like bandwidth, attenuation, and shielding effectiveness define link component design. Partners should work together closely to ensure that all critical factors are considered in connector design, to support data transmission technologies and determine whether cable or fibre is better suited for link requirements.
To achieve the possibility of Level 4 and Level 5 autonomous driving, TE Connectivity is working on next-generation data connectors that will support safety-critical, ultra-high-data volume applications such as LIDAR and RADAR, which will require speeds of up to 24 Gpbs. Currently, MATEnet and MATE-AX are available in extended performance versions that can support data speeds of 12 Gbps.
The main challenge for fully autonomous driving is enabling reliable high-speed data connectivity. Achieving this will not be easy. It will require close cooperation among partners in the automotive value chain, an industry-wide commitment to sharing best practices, and continuous technology breakthroughs. We can only achieve this vision , by working together.
By Tara Haghighi, director product management and strategy, TE Connectivity